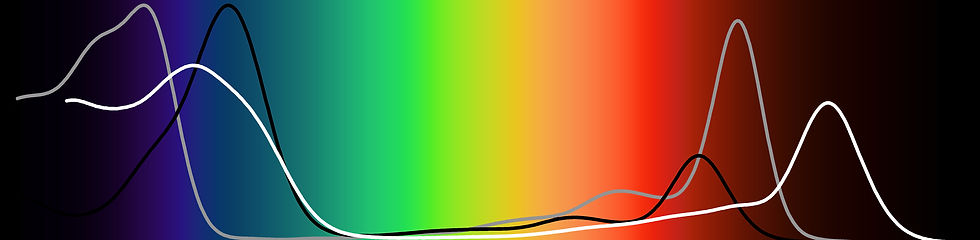
FAR-RED PHOTOSYNTHESIS
Testing the lower energy limits of photosynthetic oxygen production as a model for life around other stars.
Project Description
The search for life beyond Earth has focused on planetary bodies within our Solar System. However, the remote detection of life on planets orbiting other stars is fast becoming a reality. The Kepler mission has detected thousands of extrasolar planets, and based on this statistical sampling, researchers now estimate that there are billions of rocky exoplanets in our galaxy alone. The next step beyond detection is to characterize exoplanet habitability and then search for signs of life using ground- and space-based telescopes. Because these planets are so far away, these signs of life must be large, planetary-scale phenomena that are remotely detectable.
​
On Earth, our strongest biosignatures are from atmospheric oxygen and the vegetation red edge. The latter is a spectral reflectance feature of plant leaves that is observable through the atmosphere, manifested as absorption of most visible light between ~400–700 nm contrasting in the red with the scattering or reflectance in the near-infrared (NIR). Both of these biosignatures are a consequence of oxygen-producing (oxygenic) photosynthesis originally derived from cyanobacteria more than 2.5 billion years ago. The red wavelength of the red edge is derived from the primary oxygenic photosynthetic pigment, chlorophyll a (Chl a), present in all plants and cyanobacteria, and whose peak absorbance occurs in the red. While many anoxygenic bacteria (that is those that do not produce oxygen as a product of photosynthesis) absorb light well into the NIR, cyanobacteria have developed only two pigments to extend their peak absorption into this range, Chl d and Chl f. It has been presumed that the energetic limitations of splitting water to oxygen is a hard upper constraint for cyanobacterial absorbance.
​
The cyanobacterium Acaryochloris, with Chl d as its primary pigment, is the only known oxygenic phototroph to use a far-red light-absorbing pigment in its photosynthetic reaction centers, directly powering the conversion of water to oxygen and is, as such, of profound interest for understanding the low energy limits on this chemical conversion both on our planet and on potentially habitable exoplanets, particularly those orbiting M dwarfs that emit light primarily in the NIR. By characterizing the upper wavelength limits and limitations for Chl d-based photosynthesis in Acaryochloris, we hope to lay the foundation for constraining photosynthetic biosignatures on exoplanets orbiting cool stars, and from there generalize for any stellar temperature. This will contribute to a quantitative theoretical understanding of the energy limitation of this metabolism, which is needed to assign confidence levels to exoplanet observations seeking signs of life.
​
If you are interested in joining this exciting project, please see the advertisement here!
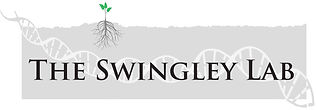